Life requires two things: a membrane that can distinguish between nothing and something, and that the “something” be an organism that is capable of reproducing.
Some time ago, American scientists created a protein that behaved just like primitive enzymes did when life evolved on Earth. This chunk of protein consisted of two chains, each of which contained 31 amino acids. The scientists also managed to produce membranes shaped like part of the bacterium.
The question is whether life could have emerged in this way, with genetic material accidentally captured inside a membranous envelope, which then led to further evolution.
The basis of all life
One thing that is certain is that super-thin membranes – which may be only a few nanometres (millionths of a millimetre) thick – are found everywhere within us and around us, acting as a sort of barrier that allows certain types of molecules to pass through, while keeping others out. And they are more important and powerful than most of us realize.
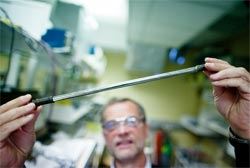
“We want clean water, clean air, food security, good use of resources and sustainable energy. That requires effective separation technology, and membranes are important,” says Rune Bredesen, as he looks at his of palladium silver membrane which can separate hydrogen from CO2.
Photo: Ståle Andersen
Click to open
The best-known is the eardrum, a thin membrane that protects the inner ear and is also set in motion by sound-induced vibrations, converting sound-waves into minute movements of the bones of the inner ear. These movements are what we perceive as sound.
Organic membranes are also essential in the world of plants. Photosynthesis, the process whereby plants use light from the Sun to convert CO2 into organic compounds such as sugars and oxygen, is one of the most important natural processes on Earth.
“The trick is to capture energy from sunlight and convert it into chemical energy,” says Trygve Brautaset, from SINTEF’s Department of Biotechnology.
“The machinery for creating energy is located inside a folded membrane in a plant cell. Here is where we find the light-sensitive parts containing chlorophyll. When sunlight activates the electrons in the molecules here, they shift into a more energetic condition, and solar energy becomes chemical energy. This is a sophisticated system with highly regulated shuttling of ions and molecules that pass through several membranes. It has been said that all higher life-forms would actually die out within 25 years if this process stopped!”
Do cell membranes control our lives?
Membranes surround every single cell in our bodies. These membranes are so fine that they were not discovered and confirmed until modern electron microscopy arrived in the late 1950s. For this reason, scientists have only recently discovered the roles played by membranes in our bodies.
Not only do membranes separate the interior of the cell from the external environment, but something in the membrane itself makes important, and correct, decisions about which substances can pass into and out of the cell. The membrane simply treats different molecules in different ways: toxic or unnecessary substances are not permitted to enter.
Cell biologist Bruce Lipton, who has taught in the schools of medicine at both the University of Wisconsin and Stanford University, has dedicated his entire career in research to cell membranes.
He claims, controversially enough, that membranes, rather than genes, are what shape our lives. It is in the membrane that the active intelligence of the cell is found. The membrane receives signals from the cell’s environment, depending on which molecules attach themselves to the membrane wall. The membrane interprets the signals and tells the cell what to do. We largely create these signals through stress, nutrition and feelings, Lipton says.
Man-made membranes
We have learned to simulate the fantastic membranes of the plant and animal world for our own purposes. Today, there are inorganic membranes virtually everywhere, whether as a permeable layer that allows most substances to pass, or as a semi-permeable layer that only allow the passage of select compounds.
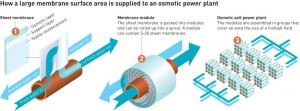
How a large membrane surface area is supplied to an osmotic power plant.
Illustration: Knut Gangåssæter
Click to open
There are inorganic membranes under the tiles on our bathroom floor, there are membranes that filter out bacteria from our drinking water and membranes that provide electricity in industrial fuel cells. Membranes are used in structures that need to withstand water pressure. They are used in gardens, under roads and in all sorts of ponds and reservoirs.
Energy and climate
SINTEF scientists Rune Bredesen and Torleif Holt have been working on membranes for several years. One researcher studies the use of membranes to improve the efficiency of CO2 scrubbing, while the other studies membranes as the basis of saline electricity generation.The world’s first prototype saline power plant was built at Hurum, outside of Oslo, in 2009. It mixes fresh-water and seawater through membranes, and is able to extract energy via osmosis. All over Europe and in the USA, teams of scientists are using membranes in saline power plants. One of SINTEF’s tasks is to help develop an optimal membrane for the Hurum plant. Thorleif Holt and his colleagues have tested hundreds of candidates, but have not reached their goal yet.
“Our membrane seems to be able to provide the cheapest and most efficient capture of CO2.”
Research Director Rune Bredesen
Now Holt is in a white coat in the laboratory and shows me a so-called sheet membrane. It looks like a glossy piece of paper. With a pair of scissors in his hands, he struggles to get the sheet to split into different layers. Finally, success, and I see the glossy thin membrane and the remaining two layers that still look like the same sheet.
“This…” he says, pointing to the membrane, “…is the layer where the osmotic process occurs. The other two layers are really just to support it.”
– What role does the membrane play in a saline power plant?
“It operates according to the same principle as in an animal cell; certain substances are allowed to pass through it, others are not. Fresh-water and saltwater are put into the same tank, separated only by a membrane. It is in the nature of the freshwater to force its way through the membrane to dilute the saltwater, which increases the pressure in the saltwater section of the tank. The freshwater passes through the membrane, but the saltwater cannot.”
Thinner and more porous
“The maximum pressure increase is comparable to a water column 250 metres high. Approximately half of this is used to drive a turbine that produces electricity.”
Holt and his colleagues have concentrated for the most part on sheet membranes. But the layers need to be made thinner and more porous, and it is important that the water is able to penetrate them. At the same time, they need to be strong.
Because an incredible amount of water per hour has to pass through an osmotic power plant, the area of membrane that is required is enormous. The membranes are packed into modules so they don’t take up too much space. Thorleif Holt shows me a sketch of a membrane module where freshwater and seawater are drawn to either side of the membranes (see figure).
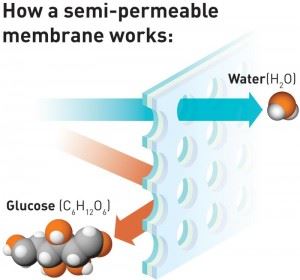
How a semi-permeable membrane works:
Illustration showing the principle behind a semi-permeable membrane. The membrane’s microscopic pores allow a water molecule (H2O) to pass, but the larger glucose molecule (C6H12O6) is too large to pass through the pores.
Illustration: Raymond Nilsson/SINTEF
Click to open
For a cleaner world
Meanwhile, Rune Bredesen and his team of scientists are working on materials designed to capture CO2. In a field that is currently regarded as one of the most important topics in applied science, membranes are an essential part of the picture.
The world is short of energy, and one method of generating electricity is by burning natural gas. The problem is that when natural gas is burned, the carbon in the gas reacts with oxygen in the air to produce CO2, which ideally should not be emitted to the atmosphere because of concerns about global warming.
The current debate regarding natural gas power stations centres on how we can lower the cost of carbon capture sufficiently to make it worthwhile to use this as a technique. Both Bredesen and his colleagues at the University of Oslo and NTNU are trying to find the cheapest and best way to do this. This means that the gas-scrubbing process must not use too much energy.
“There are many ways of scrubbing gases,” says Bredesen. “We are putting a lot of effort into removing CO2 before the gas is burnt in the power station; this is known as ‘pre-combustion technology’, where natural gas or coal is converted to hydrogen and CO2. We then use membranes to separate the two gases. When we have separated out the combustible hydrogen, we are left with CO2 that is easier to deal with, and can be stored. We use the hydrogen as fuel for electricity generation.”
– You have received a great deal of international attention because of the hydrogen membrane. Can you talk about this?
“Yes, the membrane did well in an EU project on CO2 capture that involved 24 partners. We evaluated and compared four different technologies in terms of costs and efficiency. Our membrane seems to be able to provide the cheapest and most efficient capture of CO2, and we are now working to scale up the technology. A gas-fired power plant requires several thousand square metres of membranes.”
– Why is it a good idea to use membranes?
“The conversion of natural gas to so-called synthetic gas, which is H2 and CO2, involves a lot of energy, and requires high pressures and temperatures to make it effective. Afterwards, the gas has to be cooled down before it can be separated into a liquid that captures CO2.”
With membrane technology, scientists can make both in one and the same process. In addition, they can do so at a lower temperature. The process is therefore both efficient and inexpensive at the same time, according to Bredesen. He says that the new cones consist of a thin layer of palladium-silver that only let hydrogen through. Because the layer is only about 2 / 100 mm, it sits on a porous steel tube that provides mechanical strength.
Combat global warming?
But can humanity actually control the cycles and conditions under which life exists?
The Norwegian company Albedo Technology International says yes. The company claims that its special reflector membrane could help to control the temperature of the Earth’s surface.
Between 2007 and 2009, the company carried out a series of tests in the Sahara desert, where it sprayed its product on the ground in order to create a reflective film, one that lowered the surface temperature by 45 degrees Celsius. The company tested several versions of its product on a one-million m2 test field in Morocco, and climate scientists at Norway’s Cicero climate research centre confirmed that the biomembrane has great potential if it can be made to stay in place on large areas for long periods of time.
The company is now about to start a parallel research project in the Arizona desert in the USA, in a wide-ranging cooperative programme that will document what sort of changes take place when large amounts of energy are reflected back into space from the Earth’s surface.
It remains to be seen whether the promise of this bio-membrane will come true. In any case, we may ask whether the “original” biological membranes, in the course of their faithful everyday efforts to keep our bodies and life on Earth in general going, are still the most advanced available.
Åse Dragland